— What synchronizes the development of the opposing body sides in symmetrical organisms?
— Does the existence of biological symmetry prove the intelligent design?
— What’s a “mirror gene”, and how does it work?
Biological life excels at symmetry. Sculptors and artists struggle so much to pull it off in their work: years of practice, discipline and learning – and yet billions of things are born on this planet every hour that somehow come symmetrical out of the box.
There must be a secret to it. A symmetry lifehack hidden in our genes:
- Perhaps, a mirror gene that works like a “flip horizontal” button in PhotoShop™;
- Or some proofreading biochemical mechanism that collates the two sides of the developing organims;
- Or is it just ruthless natural selection that eliminates anyone whose genes for the left and right sides are anything put perfectly identical?
It’s neither actually. As counterintuitive as it may sound, life doesn’t need any lifehacks to create symmetry, because biological symmetry is the easiest and the dumbest feature in the entire embryogenesis.
Symmetry may take effort to achieve in sculpture and art – but in developmental biology, symmetry is actually the default (!) condition.
Spherical symmetry
In presense of intacellular adhesion, any colony of cells will automatically arrange itself into the spherical shape (unless it’s constrained by some external mechanical factors).
It happens for the same reason that there are no cubic planets: any group of particles bound together by forces of attraction will always become round to reduce its potential energy.
Except… what’s it have to do with symmetry?
Everything! Of all geometric shapes, it’s the sphere that has the most perfect symmetry: the only shape that can be cut in half at any angle and in any plane and render two equal halves.
It’s called spherical symmetry: when an object mirrors across all three planes of the 3D-space.
Turns out that perfect symmetry in all directions and planes is the default condition of multicellular organisms. No need for any special genes or divine interventions, because physics 101 does all the job. No separate gene sets for different sides either, because the cells from all sides just express the SAME genes: when one side evolves, so do the other sides, automatically.
A colony of cells expressing the same genes and condensing into the most stable shape in the universe – no big deal. It’s making a colony triangular, skewed, or Apple-shaped™ that’s the trick.
Q: Which organisms have spherical symmetry?
Adult organisms – surprisingly few: mostly some unicellular organisms and collonial algae. Which doesn’t make it rare though, because it’s present in the majority of organisms at the earliest stages of development:
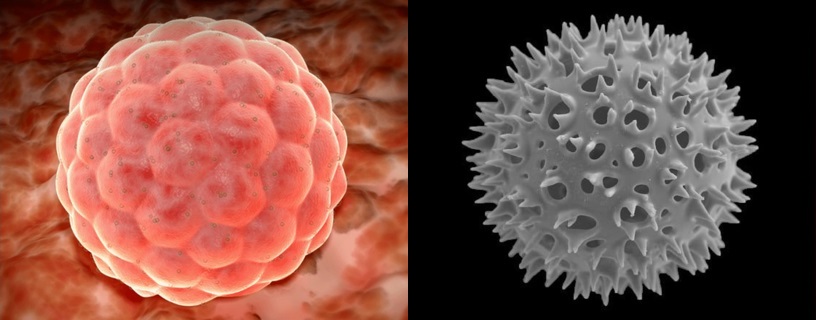
Recognize yourself on the image to the left? And the right one is the mineral skeleton of a radiolarian.
Spherical symmetry is useful because it allows organisms to “act” identically in all directions regardless of orientation in space. For example, it’s crucial for volvox (algae colonies bobbing about in water) that the efficiency of photosynthesis stays the same regardless of how it’s oriented relative to the sun.
But in the end, spherical symmetry doesn’t have to be useful to exist, because it’s the baseline. You don’t need a good reason to be spherical – you need a good reason to evolve out of it.
Radial symmetry
Radial symmetry is the second-most perfect symmetry after spherical. Unlike the salamander embryo, radial creatures preserve symmetry in two directions (left-to-right and front-to-back), but differentiate vertically.
A big mistake is to think that “less symmetry = less complexity”. In actuality, the radial body plan is more complex than the spherical one precisely because it’s less symmetrical: breaking that symmetry along one of the axes, forcing different groups of cells into following different developmental paths – that’s where the real complexity begins.
And that’s exactly what makes an actinian more fun than a boring round blob of cells:
To break the symmetry, something has to disrupt the uniformity of cells at the top and bottom poles of the embryo: to follow different developmental paths, the cells themselves must be physically different from each other in some way (for example, in chemical composition).
Usually, it involves a special substance called morphogen that distributes unevenly thoughout the cell and forms a gradient. After the cell divides, its daughter cells end up with different amounts of the morphogen and start expressing different genes because of that:
There are several ways in which the gradient can be created:
- Gravity – when the morphogen sinks to the bottom of the cell under its own weight.
- Fertilization – when the egg is chemically modified by the sperm specifically at the point of their fusion (e.g., the point of sperm entry will become the top of the embryo).
- Maternal factors – when the mother places the morphogen inside the egg at one of its poles during oogenesis.
An embryo needs a chemical guide to develop radial symmetry: something to tell where the vertical body axis is and where it points. It took hundreds of millions of years for life to polish this mechanism and master consistent differentiation in one axis.
Q: Why would radial organisms “intentionally” sacrifice spherical perfection and ditch symmetry in one axis?
Remember the advantages of spherical symmetry: acting in all directions equally and with the same efficiency, regardless the orientation in space?
So, what if an organism needs to act differently in two directions: for example, to catch food with one end of the body and crawl on the floor with the other end? What if an organism has a stable orientation in space: for example, it spends its whole life sitting in the mud or attached to substrate?
In those cases, vertical differentiation becomes advantageous: a body part good at crawling or attaching doesn’t have to be good at catching food, and vice versa. So why not develop two different body parts and place them at the opposite ends of the body: one part will catch food, the other crawl, and they won’t interfere with each other?
That’s why many sessile creatures are radially-symmetrical: they use their bottoms to cling onto substrate and their tops to catch food. For example, a freshwater hydra:
The two ends of its body are clearly distinct: the bottom forms a sticky foot to grasp onto twigs, and the top is armed with tentacles around the mouth.
Another example is a star fish:
Starfish are benthic animals that crawl on the ocean floor for food. Not unexpectedly, their mouths and walking legs are all on the underside, while their top sides are tailored for other purposes (defence, camouflage etc).
Essentially, asymmetry is a form of specialization: organisms sacrifice the ability to do everything in all directions and optimize different ends of the body for different tasks.
Note that radials preserve horizontal symmetry: a starfish can equally crawl in any direction, while a hydra is good at catching prey approaching from any side. As far as they can tell, there is no left/right/front/back: there is only bottom, top, and “aside” – which is 360 degrees around.
Bilateral symmetry
Bilateral symmetry is the most imperfect of all symmetries. While hydras and jellyfish are differentiated across a single plane, crayfish, worms and dogs are all differentiated across two planes at the same time: you can easily tell their fronts from their rears (in addition to top from bottom).
Left-to-right symmetry is all they got left:
Breaking symmetry in one direction is difficult enough, but breaking it in two at a time isn’t just twice as hard, but more like twenty times! Not only two independent inciting events have to establish two gradients of two different morphogens in the embryo – but they also must do it perfectly perpendicular to each other.
Just sticking two morphogens into a cell at two random places won’t do: if the two gradients accidentally align, the belly and the butt might end up being the same place (whatever that means).
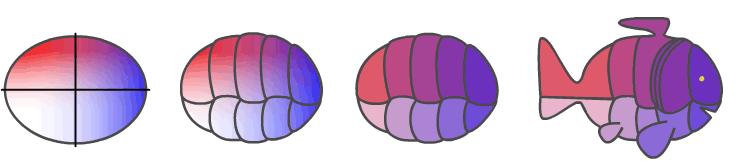
As one wise man once said: “What a fuck was that?”
Bilateral body plan is indeed marvelous, except most people marvel at the wrong thing. It’s not left-to-right symmetry that makes us interesting and aesthetic, but the lack of symmetry in every direction except left-to-right. It’s breaking symmetry along two axes that takes months in the womb, complex developmental pathways, and thousands of genes: all to prevent cells at the opposing sides from following the same fates and becoming the mirrored images of each other.
In developmental biology, asymmetry is and always has been the real magic. Counterintuitive indeed.
Q: Elaborate on the motivation thing.
Bilaterality is the adaptation for active locomotion: instead of being able to slowly crawl in any direction, bilaterals adapted to move only forward, but very quickly and efficiently. That’s why the horizontal differentiation: to move face forward, you need a face.
Essentially, the fish above sacrifices the power to swim backwards or sideways in order to excel at swimming forward.
Q: You need front-to-back differentiation to swim forward, but why top-to-bottom? Couldn’t fish just turn the radial body plan sideways to swim horizontally in one direction? Why are back and belly necessary for active locomotion?
Because up and down are fundamentally distinct on Earth:
- It takes effort to go up, while everything sinks down on its own
- There is the sun up there, while down there… there’s no sun
- There is ground or bottom below that one can crawl or dig into, while above there’s none unless the firmament counts
It’s just the way nature is: the belly and the back of an animal are affected by different factors. There’s gravity that pulls downwards, there are predators that always strike from above, there’s food that can be picked from the bottom etc.
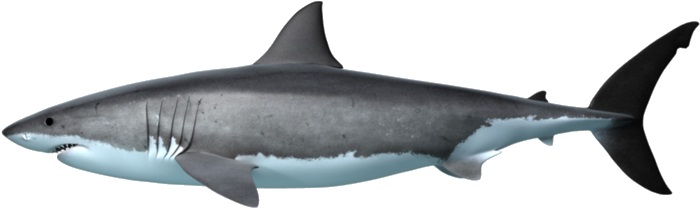
1) Upper vs lower fin asymmetry negates the gravitational pull when swimming; 2) Mouth located at the underside of the head, because biting down on the prey is more effective; 3) Back and belly colours are different for camouflage (the darker back blends with the ocean deep when seen from above, and the white belly blends with the sunlight when seen from below).
Same for terrestrial animals: walking with feet on the floor and on the ceiling simultaneously – even in horror movies they either do one or the other, but never both at the same time.
In other words, the upper and lower parts of animals are subject to different selective pressures, and pretty much evolve independently from each other.
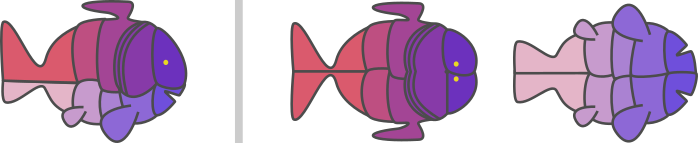
For clarity, a normal fish vs two fishes that decided to skip the vertical differentiation day (all three pictures are the side views).
So why would left and right stay the same? Simple: because as far as any creature is concerned, there is no fundamental distinction between the two, not to mention that they are relative in the first place (a quick 180° turn, and everything that used to be to the left is suddenly to the right). There are no environmental factors that consistently affect creatures from one of the two sides: no predators that bite their prey only on the left side, or winds that always (magically) blow from the right.
Furthermore, effective locomotion requires even force application, which means that an asymmetrical fish wouldn’t be able to swim straight. Make the left pectoral fin of a fish different from the right one, and when it tries to swim ahead it will end up anywhere but ahead. Physics 101 creates the symmetry – physics 101 punishes those who don’t respect it.
Conclusion
People are so used to symmetry being something difficult and complex that they inevitably project those views onto living organisms. It’s true: painting a symmetrical picture, sculpting a symmetrical sculpture, and manufacturing symmetrical furniture takes skill and dedication.
However, living organisms aren’t made of wood or clay, and are shaped through fundamentally different natural processes. They don’t rely on divine interventions, mirror genes or quantum protein reflectors for symmetry – those aren’t necessary. Nothing can be simpler than a bunch of cells forming a symmetrical shape through adhesion and then maintaining that shape through identical gene expression.
Not only symmetrical magic doesn’t exist – there is no room to apply it even if it did.
P.S. Meanwhile, asymmetry is a true art.
Q: If symmetry is the default, why do we have asymmetrical internal organs? How could it be the default when it’s just superficial?
Because internal asymmetry is a progressive feature.
The developmental program of some creatures is so complex that it’s capable of producing overall bilaterality but with some asymmetrical elements. Which means that they differentiate not along two, but three (!) planes simultaneously, thus breaking the symmetry in every possible direction!
Like our digestive system:
Such differentiation is the pinnacle of developmental complexity, taking hundreds of morphogens, complex cellular migration pathways, signal molecules etc. We can tell that our internal asymmetry isn’t just some accident or archaism, because the layout is inheritable and consistent from individual to individual: all people have their livers to the right, stomachs to the left, and colons curving the same way. Meaning that there are developmental mechanisms in place that coordinate their locations inside the body: our livers don’t float randomly in the belly, but consistently happen in the exact same place from generation to generation.
Furthermore, we can tell that it’s a progressive feature because basal vertebrates are internally nearly symmetrical, not to mention that human embryos start internally symmetrical and break from it later.
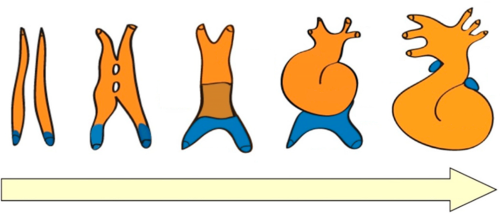
© Jaconi & Puceat The development of heart, the first organ to lose the left-to-right symmetry.
Q: If symmetry is the default, why are there so many asymmetrical organisms: sponges, most plants, mushrooms etc?
All creatures on the list possess the default radial symmetry: a tree is obviously differentiated vertically into a root, trunk and crown; a sponge is differentiated into a base and an osculum; and a mushroom – into a stalk and a cap. Meanwhile, all three aren’t horizontally differentiated at all.
Which begs the question: how can they be “technically” radial without actual radial symmetry?
The answer: because they can’t keep it. They grow and differentiate for life, under the influence of many environmental factors that affect the growth of their different parts. For example, trees will “reach” towards the light, while sponges will “bend” under the ocean currents. Uneven lighting, winds, currents and substrate – all those act as thousands of “micro-morphogens” that enhance, silence, or redirect the growth of separate twigs or branches, which in the end distorts the overall symmetry.
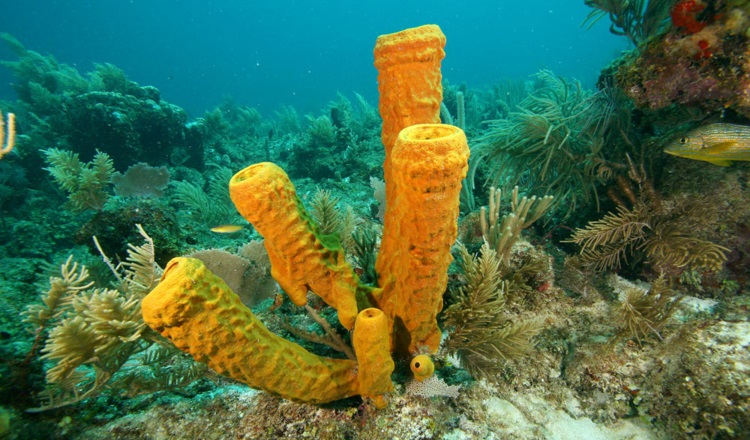
People like to bring up sponges as an example of asymmetry, while their original radiality is very much legible.
On the other hand, plant a tree inside a green house with perfectly even conditions: uniform lighting from all sides, uniform nutrient and water distribution in the soil etc – the tree will end up perfectly symmetrical.
In a way, trees and sponges illustrate the main gist of this article: “Asymmetry is always the symmetry that’s been broken or distorted by something”.
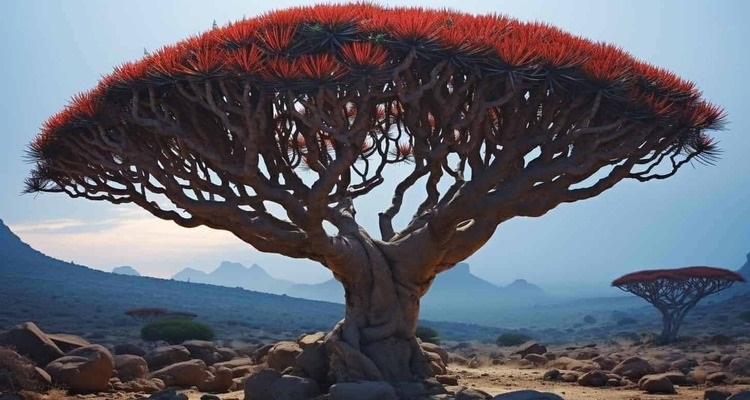
Despite its asymmetry, dragon blood tree obviously has underlying radial symmetry that’s been tweaked here and there by the environmental factors.
Q: Why does it not happen to us?
Unlike plants, corals and sponges, our bodies are shaped almost entirely during the dedicated emryonic stage of development that takes place in protected, uniform environment (womb/egg/etc), sheltered well from external pressures. By the time we are born, our overall body plan is already in place, and environmental factors largely cannot affect it.
Basicallly, the perfect symmetry recipe is to give a colony of cells some intracellular adhesion, but them into a uniform environment, and disturb as little as possible.
About morphogenesis
Symmetry is a response to mechanical forces